Author(s): Eleanor M.Mullen, DanielAnkeny
- Cerebral blood flow (CBF) is equal to cerebral perfusion pressure (CPP) divided by the cerebral vascular resistance. CPP is defined as the difference between the mean arterial pressure (MAP) and intracranial pressure (ICP) or central venous pressure, whichever is higher. CBF averages 50 mL/100 g of brain tissue per minute in the normal brain and is affected by blood pressure, metabolic demands, PaCO2, PaO2, blood viscosity, vasoactive agents, and neurogenic regulation. The brain receives approximately 15% of the cardiac output.
- CBF is maintained at a constant level by constriction and dilation of arterioles (autoregulation) (Figure 23.1) when the MAP is between 50 and 150 mm Hg. This concept is known as cerebral autoregulation. When MAP is outside these limits, CBF varies directly with the MAP. Chronic hypertension shifts the autoregulatory curve to the right, rendering hypertensive patients susceptible to cerebral ischemia at blood pressures considered normal in healthy individuals. Chronic antihypertensive therapy likely normalizes the autoregulatory range. Cerebral ischemia, trauma, hypoxia, hypercarbia, edema, mass effect, and volatile anesthetics attenuate or abolish autoregulation and may make blood flow to the affected area dependent on MAP.
Figure 23-1 Autoregulation maintains a constant level of cerebral blood flow (CBF) over a wide range of carotid artery mean blood pressures.
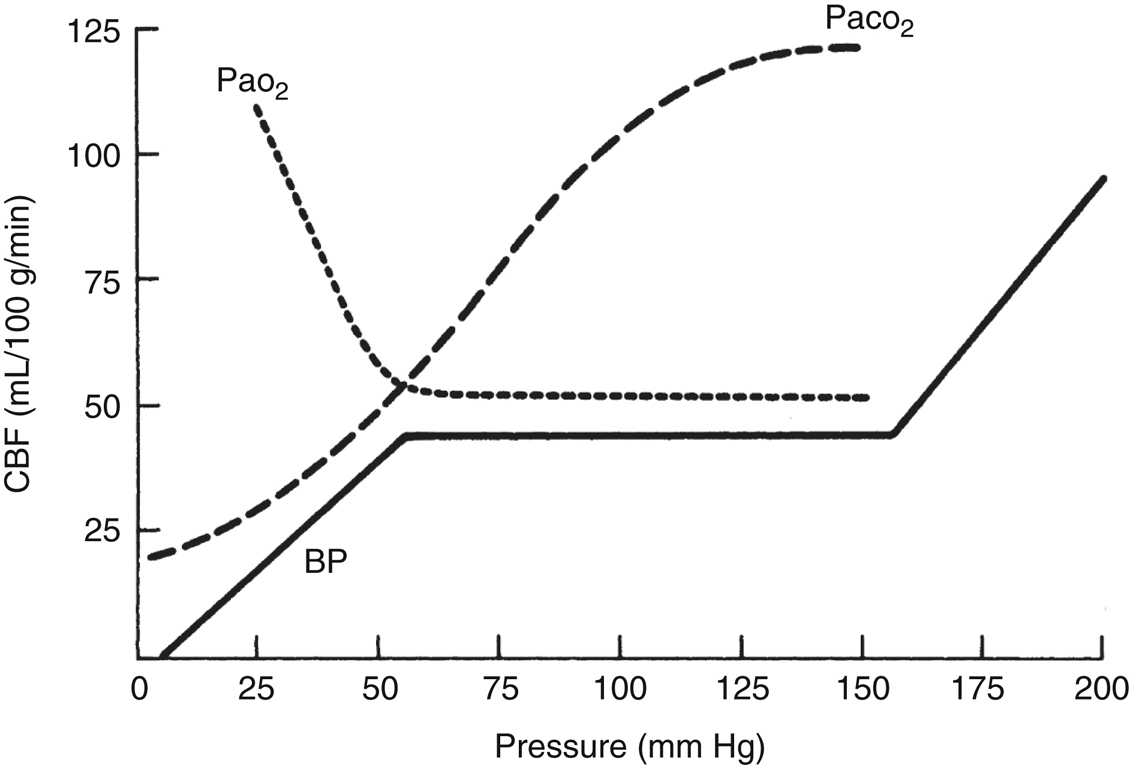
Independent of this effect, CBF is elevated by hypercarbia (PaCO2) and hypoxemia (PaO2); hypocarbia diminishes CBF.
- PaCO2 has profound effects on CBF by its effect on the pH of the brain extracellular fluid (ECF). CBF increases linearly with increasing PaCO2 in the range from 20 to 80 mm Hg, with an absolute change of 1 to 2 mL/100 g/min for each mm Hg change in PaCO2. The effect of PaCO2 on CBF decreases over 6 to 24 hours because of slow adaptive changes in the brain ECF bicarbonate concentration. Sustained hyperventilation causes cerebrospinal fluid (CSF) bicarbonate production to decrease, allowing CSF pH to gradually normalize. Rapid normalization of PaCO2 after a period of hyperventilation results in a significant CSF acidosis with vasodilation and increased ICP.
- PaO2. Hypoxia is a potent cerebral vasodilator. CBF increases markedly below a PaO2 of 60 mm Hg. PaO2 above 60 mm Hg has little influence on CBF. However, hyperoxia may be deleterious under conditions of neuropathology, via promotion of oxidative injury.
- Neurogenic regulation. The cerebral vasculature receives extensive cholinergic, adrenergic, serotonergic, and VIPergic innervation, though the exact role these systems play in regulation of CBF is not clear. Evidence suggests, however, that increased sympathetic tone in hemorrhagic shock shifts the lower end of the autoregulatory curve to the right and results in lower CBF at a given MAP.
- Viscosity. Normal hematocrit (33%-45%) in a healthy brain has little influence on CBF. During focal cerebral ischemia, however, reduction in viscosity by hemodilution (hematocrit 30%-34%) may increase CBF to ischemic territories.
- Cerebral metabolic rate (CMRO2) and CBF are tightly coupled because the brain requires a constant supply of energy to meet its high metabolic demands. Regional or global increases in CMRO2 elicit a corresponding increase in CBF, probably mediated by signaling molecules such as nitric oxide. Other factors that modulate CMRO2 (and CBF through this mechanism) include the following:
- Anesthetics. Variable effect (Sections II.A and B).
- Temperature. Hypothermia decreases CMRO2 by 7% per 1 °C below 37 °C, while hyperthermia increases CMRO2.
- Seizures. Increases CMRO2.
- Pain or arousal. Increases CMRO2.
- ICP reflects the relationship between the volume of the intracranial contents (brain, blood, and CSF) and the volume of the cranial vault. Normal ICP varies between 5 and 15 mm Hg. A sustained elevation of ICP greater than 15 to 20 mm Hg in the setting of intracranial pathology is considered abnormal.
- The cranial vault is rigid, and its capacity to accommodate increases in intracranial volume is limited. A developing intracranial mass (eg, tumor, edema, hematoma, or hydrocephalus) initially displaces blood and CSF, and ICP remains relatively normal (Figure 23.2). As intracranial volume increases further, intracranial compliance decreases, and ICP rises rapidly (Figure 23.2). Thus, patients with decreased compliance may develop marked increases in ICP even with small increases in intracranial volume (eg, cerebral vasodilation due to anesthesia, hypertension, or carbon dioxide retention) (Figure 23.2).
Figure 23-2 The intracranial compliance curve.
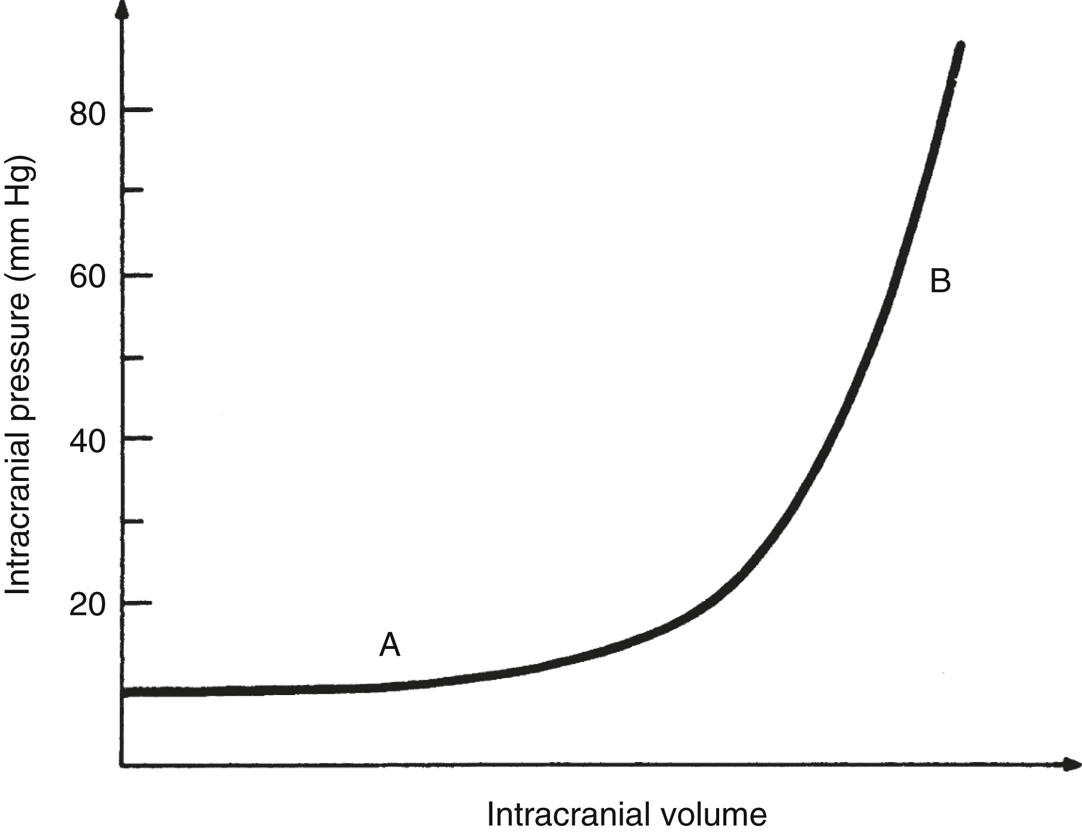
In the normal intracranial pressure (ICP) range (A), increases in intracranial volume produce minimal changes in ICP. Further, small increases in ICP after the elbow of the curve can produce abrupt increases in ICP (B).
- Clinical features of elevated ICP. ICP elevation usually decreases CPP and may cause ischemia in regions of the brain where autoregulation is defective and CBF depends on CPP. Early signs and symptoms of increased ICP include headache, nausea, vomiting, blurred vision, papilledema, and decreased levels of consciousness. As ICP increases, brain herniation may occur causing mechanical injury and/or ischemia to the brainstem and cranial nerves. This may result in hypertension with bradycardia or tachydysrhythmia, irregular respiration, oculomotor (third cranial nerve) palsy leading to ipsilateral pupillary dilation with absent light reflex, abducens (sixth cranial nerve) palsy, contralateral hemiparesis or hemiplegia, and ultimately, coma and respiratory arrest.
- Treatment of elevated ICP involves strategies aimed at decreasing the volume of the intracranial components, as outlined below:
- Hyperventilation to a PaCO2of 25 to 30 mm Hg produces cerebral vasoconstriction and may be used as a temporizing measure in the management of acutely increased ICP. Hyperventilation is potentially deleterious, however, and can cause ischemia in injured brain and penumbra where CBF is low. Therefore, hyperventilation should be withdrawn when effective definite therapy is established. Moreover, the effect of hyperventilation on ICP decreases as CSF pH normalizes. Conversely, hypoxia and hypercarbia cause cerebral vasodilation and should be avoided.
- Decrease jugular venous pressure. Elevating the head at least 30° from supine promotes venous drainage and decreases intracranial venous blood volume, thereby lowering ICP. Avoid excessive flexion or rotation of the neck and prevent increases in the intrathoracic pressure (eg, coughing, straining, and elevated intrathoracic pressure), since each of these may decrease jugular outflow. Similarly, positive end-expiratory pressure should be minimized to the lowest level that maintains adequate lung recruitment.
- Control CMRO2. Barbiturates are potent vasoconstrictors that decrease cerebral blood volume while decreasing CMRO2. Prevent increases in CMOR2 due to arousal/seizures with adequate sedation and seizure prophylaxis where indicated.
- Maintaining high serum osmolality (305-320 mOsm/kg) may reduce cerebral edema and decrease brain volume via promotion of favorable fluid gradients (hydrostatic and oncotic pressures). Fluid management is designed to achieve this goal (Section V.D). In addition, mannitol (0.5-1.0 g/kg intravenous [IV]) and furosemide produce a hyperosmolar state and are effective in the acute reduction of ICP. Hypertonic saline is an alternative to mannitol for managing raised ICP.
- CSF volume can be reduced by draining the CSF through ventriculostomy catheter or needle aspiration intraoperatively, or even preoperatively when ICP is dangerously elevated.
- Surgical removal of tumor, hematoma, or decompressive craniectomy reduces intracranial volume and ICP.
- Steroids may reduce cerebral edema associated with tumors, though this is a gradual process and likely does not contribute to acute ICP management.