Description- The blood oxygen-carrying capacity is the milliliters of oxygen present in 1 deciliter of blood.
- In blood, oxygen can exist in 2 forms: Dissolved and attached to hemoglobin. Hemoglobin is the primary transporter of oxygen in mammals.
- In the event of decreased oxygen delivery, the blood provides a limited cushion against hypoxia.
- Oxygen is necessary for aerobic metabolism and cell integrity. However, despite its absolute necessity, the body only has minimal storage capacity (unlike glucose). When apneic, oxygen stores are limited to the oxygen in the lungs (FRC) and the blood; both of which are unable to sustain life beyond a few minutes.
- In a healthy adult at rest, oxygen consumption is ~200250 mL O2/min and oxygen delivery is ~9501,150 mL O2/min. Thus, ~25% of the arterial oxygen is used every minute, and the deoxygenated blood returning to the lungs has ~75% saturation (mixed venous oxygen saturation) (1).
- Hemoglobin provides the most efficient means of carrying and transporting blood. At full oxygen saturation, 1 g of hemoglobin in 1 dL of blood is capable of carrying 1.34 mL of O2. Thus, Hgb × 1.34 × SaO2 determines the milliliters of oxygen bound to hemoglobin in 1 dL of blood. Oxygen bound to hemoglobin does not exert partial pressure.
- Oxygen is poorly soluble in blood with a coefficient of 0.003 milliliters of oxygen per deciliter of plasma per millimeter of mercury oxygen. Hence, a patient breathing room air with an approximate PaO2 of 100 mm Hg has 0.3 mL of oxygen dissolved in 1 dL of blood.
- Oxygenhemoglobin (O2Hgb) dissociation curve: PaO2 on the x-axis, and SaO2 on the y-axis. As PaO2 increases, SaO2 increases. The curve is not linear, but sigmoid in shape. This represents hemoglobin's desire to be fully bound or fully dissociated from oxygen; it is unstable in intermediate states. This characteristic facilitates its physiologic function; at the lungs, hemoglobin will fully saturate and at the tissue level, it will easily dissociate and unload to oxygenate the tissues.
- Assuming normal hemoglobin moieties and conditions, the PaO2 is in equilibrium with oxyhemoglobin and dissolved oxygen. for example, a PaO2 of 100 mm Hg has an SpO2 of 98% and 0.3 mL O2/dL of blood. A PaO2 of 500 mm Hg has an SpO2 of 100% and 1.5 mL O2/dL of blood.
- Hemoglobin is an iron-containing protein that is composed of 4 polypeptide chains or subunits. The iron-containing heme group of each polypeptide reversibly binds up to 4 molecules of oxygen (2).
- The adult hemoglobin (HbA) molecule is made of 2
globin and 2
globin subunits. - The binding of the first oxygen molecule to hemoglobin induces conformational changes in the structure of the hemoglobin that allows the binding of other molecules of oxygen more readily. At normal resting conditions, 25% of the oxygen bound to hemoglobin is extracted by the tissues (equivalent to a partial pressure of 40 mm Hg).
- Fetal hemoglobin (HbF), on the other hand, has 2
and 2
subunits that possess a strong affinity for oxygen. The fetus is exposed to much lower oxygen pressures from the placenta; ~21% of the level found in the adult lung (1).
Physiology/Pathophysiology- Low oxygen-carrying capacity is the result of a low PaO2, low hemoglobin, impaired oxygen-to-hemoglobin binding, or an increased oxygen demand/consumption.
- Low PaO2: Hypoxic mixture (low inspired oxygen), hypoventilation, V/Q mismatching, shunting, impaired diffusion
- Low hemoglobin: Chronic or acute anemia
- Impaired oxygen-to-hemoglobin binding: Abnormal hemoglobins (fetal, thalassemia, sickle cell disease), carboxyhemoglobin, cyanhemoglobin, methemoglobin, sulfhemoglobin
- Increased oxygen demand/consumption: Hyperthermia, hyperthyroidism, sepsis, pregnancy, vigorous exercise
- Acute anemia: The initial hemodynamic response is a fall in systemic vascular resistance (SVR) that is partly due to the decrease in blood viscosity and in part, the result of nitric oxide-mediated vasodilation. The decrease in SVR reduces blood pressure and causes a baroreceptor-mediated neurohormonal activation, identical to that seen in patients with severe low-output heart failure. Eventually, sympathetic and reninangiotensinaldosterone activity cause peripheral vasoconstriction and decrease in renal blood flow and glomerular filtration rate. Kidneys retain salt and water (3).
- Chronic anemia results in hemodynamic and non-hemodynamic (erythropoiesis) compensatory responses to enhance oxygen-carrying capacity (2,4).
- Hemodynamic responses are complex and involve a vasodilation-mediated high-output state with neurohormonal activation (as described above in acute anemia). The high-output state initially helps to increase oxygen transport. However, compensatory mechanisms have deleterious long-term consequences and could contribute to anemia's role as an independent risk factor for adverse outcomes.
- Non hemodynamic responses: Increased levels of 2,3-diphosphoglycerate (2,3-DPG) shift the O2Hgb dissociation curve to the right. This is the least energy-consuming mechanism to support increased oxygen delivery to the tissues (lacks significant increase in cardiac output). Erythropoiesis also serves to increase red blood cell production.
- In elevated altitudes, ventilation and heart rate are elevated with a minimum reduction in stroke volume. In addition, plasma volume is reduced over 2448 hours to improve the oxygen-carrying capacity of the blood. Prolonged sojourn at altitudes is compensated for with enhanced erythropoiesis and a larger hemoglobin mass, allowing for a partial or full restoration of the blood volume and arterial oxygen content.
- General anesthesia causes a 15% reduction in metabolic rate and a subsequent decrease in oxygen requirement. Artificial ventilation further decreases the oxygen requirement by 6% with the removal of the work of breathing. Anesthetic agents, however, do not affect the transport of oxygen by hemoglobin or its solubility in blood.
- The increased utilization of oxygen when metabolic rate is increased (postoperative shivering, malignant hyperthermia, etc.) leads to a decrease in PaO2 which activates normal protective responses to hypoxia (aortic and carotid chemoreceptors, sympathetic nervous system) to increase cardiac output. However, these protective responses are usually reduced by anesthetic drugs intraoperatively, and can extend into the postoperative period.
- Blood transfusion: It remains the clinician's responsibility to determine the transfusion "trigger" for an individual patient based on multiple elements that determine the demand for the delivery of oxygen and the physiological reserve. The decision should be based on the premise that the oxygen-carrying capacity is increased to prevent oxygen consumption from exceeding oxygen delivery. Additionally, the following should be considered:
- Theoretically, the oxygen-carrying benefit of red blood cells should hasten recovery from respiratory failure, and transfusion would therefore be expected to shorten the duration of mechanical ventilation. However, evidence to the contrary has been reported.
- Storage of packed red blood cells (pRBCs) is associated with a decline in 2,3-DPG that leads to a subsequent left shift of the O2Hgb dissociation curve. Therefore, while transfusion increases the patient's hemoglobin, it results in a less efficient oxygen delivery when compared to the native hemoglobin at that same hematocrit. 2,3-DPG levels return to normal over 1224 hours.
- The need to restore blood viscosity may precede the need to restore oxygen-carrying capacity during hemodilution or anemic conditions. A minimum level of blood viscosity appears to be necessary for generating shear stress and stimulating the release of vasoregulatory factors such as nitric oxide and prostacyclin (3).
- Studies in subpopulations (renal failure, Jehovah's Witnesses, military casualties) have shown that considerably greater amounts of anemia than was previously believed can be well tolerated.
- Patients that are unable to increase their cardiac output (coronary artery disease, prior/acute myocardial infarction, beta-adrenergic blockade, decreased SVR such as sepsis or post-cardiopulmonary bypass) or have impaired oxygenation (pulmonary disease, high altitude) have limited compensatory responses to hypoxia.
- Isovolemic hemodilution to hemoglobin levels of 5 g/dL in normal volunteers is well tolerated. A robust cardiovascular response manifested by increases in heart rate and stroke volume leads to compensation.
- Blood substitutes are being investigated to increase oxygen-carrying capacity. At this time, there are no available products for clinical use.
- Oxygen content = [(Hgb × 1.39 × SaO2) + (PaO2 × 0.003)]. Units are as follows: Hgb is in g/dL; 1.39 is mLO2/dL; SaO2 is % (0.01) but no units (5).
- Oxygen arterial content (CaO2) = [(Hgb × 1.39 × SaO2) + (PaO2 × 0.003)] (5).
- Oxygen venous content (CvO2) = [(Hgb × 1.39 × SvO2) + (PvO2 × 0.003)]. If obtained at the pulmonary artery (PAC), it is a representation of the true mixed venous oxygen (5).
- Oxygen delivery (DO2) (mL/min) = cardiac output (CO) × blood oxygen content
Oxygenhemoglobin dissociation curve
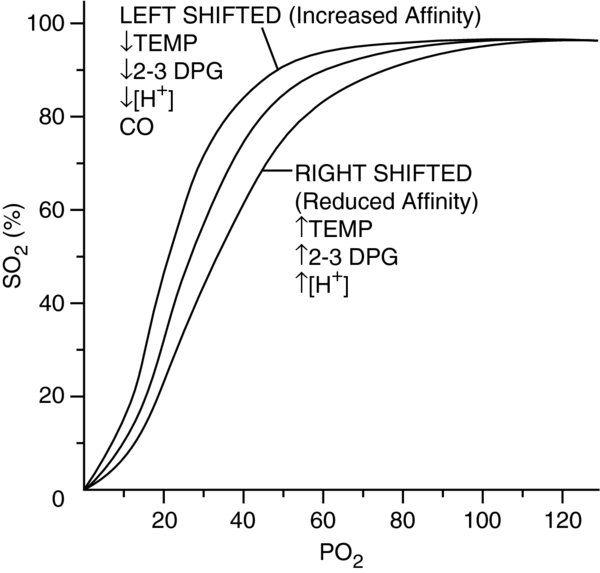